How Old is the Universe?
Until recently, astronomers estimated that the Big Bang occurred between 12 and 14 billion years ago. To put this in perspective, the Solar System is thought to be 4.5 billion years old and humans have existed as a genus for only a few million years. Astronomers estimate the age of the universe in two ways: 1) by looking for the oldest stars; and 2) by measuring the rate of expansion of the universe and extrapolating back to the Big Bang; just as crime detectives can trace the origin of a bullet from the holes in a wall.
OLDER THAN THE OLDEST STARS?
Astronomers can place a lower limit to the age of the universe by studying globular clusters. Globular clusters are a dense collection of roughly a million stars. Stellar densities near the center of the globular cluster are enormous. If we lived near the center of one, there would be several hundred thousand stars closer to us than Proxima Centauri, the star nearest to the Sun.
Text Link to the HST press release describing this image
The life cycle of a star depends upon its mass. High mass stars are much brighter than low mass stars, thus they rapidly burn through their supply of hydrogen fuel. A star like the Sun has enough fuel in its core to burn at its current brightness for approximately 9 billion years. A star that is twice as massive as the Sun will burn through its fuel supply in only 800 million years. A 10 solar mass star, a star that is 10 times more massive than the Sun, burns nearly a thousand times brighter and has only a 20 million year fuel supply. Conversely, a star that is half as massive as the Sun burns slowly enough for its fuel to last more than 20 billion years.
All of the stars in a globular cluster formed at roughly the same time, thus they can serve as cosmic clocks. If a globular cluster is more than 20 million years old, then all of its hydrogen burning stars will be less massive than 10 solar masses. This implies that no individual hydrogen burning star will be more than 1000 times brighter than the Sun. If a globular cluster is more than 2 billion years old, then there will be no hydrogen-burning star more massive than 2 solar masses.
The oldest globular clusters contain only stars less massive than 0.7 solar masses. These low mass stars are much dimmer than the Sun. This observation suggests that the oldest globular clusters are between 11 and 18 billion years old. The uncertainty in this estimate is due to the difficulty in determining the exact distance to a globular cluster (hence, an uncertainty in the brightness (and mass) of the stars in the cluster). Another source of uncertainty in this estimate lies in our ignorance of some of the finer details of stellar evolution. Presumably, the universe itself is at least as old as the oldest globular clusters that reside in it.
EXTRAPOLATING BACK TO THE BIG BANG
An alternative approach to estimating is the age of the universe is to measure the “Hubble constant”. The Hubble constant is a measure of the current expansion rate of the universe. Cosmologists use this measurement to extrapolate back to the Big Bang. This extrapolation depends on the history of the expansion rate which in turn depends on the current density of the universe and on the composition of the universe.
If the universe is flat and composed mostly of matter, then the age of the universe is
2/(3 Ho)
where Ho is the value of the Hubble constant.
If the universe has a very low density of matter, then its extrapolated age is larger:
1/Ho
If the universe contains a form of matter similar to the cosmological constant, then the inferred age can be even larger.
Many astronomers are working hard to measure the Hubble constant using a variety of different techniques. Until recently, the best estimates ranged from 65 km/sec/Megaparsec to 80 km/sec/Megaparsec, with the best value being about 72 km/sec/Megaparsec. In more familiar units, astronomers believe that 1/Ho is between 12 and 14 billion years.
AN AGE CRISIS?
If we compare the two age determinations, there is a potential crisis. If the universe is flat, and dominated by ordinary or dark matter, the age of the universe as inferred from the Hubble constant would be about 9 billion years. The age of the universe would be shorter than the age of oldest stars. This contradiction implies that either 1) our measurement of the Hubble constant is incorrect, 2) the Big Bang theory is incorrect or 3) that we need a form of matter like a cosmological constant that implies an older age for a given observed expansion rate.
Some astronomers believe that this crisis will pass as soon as measurements improve. If the astronomers who have measured the smaller values of the Hubble constant are correct, and if the smaller estimates of globular cluster ages are also correct, then all is well for the Big Bang theory, even without a cosmological constant.
WMAP CAN MEASURE THE AGE OF THE UNIVERSE
Measurements by the WMAP satellite can help resolve this crisis. If current ideas about the origin of large-scale structure are correct, then the detailed structure of thecosmic microwave background fluctuations will depend on the current density of the universe, the composition of the universe and its expansion rate. WMAP has been able to determine these parameters with an accuracy of better than than 3% of the critical density. In turn, knowing the composition with this precision, we can estimate the age of the universe to about 1%: 13.7 ± 0.13 billion years!
How does WMAP data enable us to determine the age of the universe is 13.7 billion years, with an uncertainty of 1%? The key to this is that by knowing the composition of matter and energy density in the universe, we can use Einstein's General Relativity to compute how fast the universe has been expanding in the past. With that information, we can turn the clock back and determine when the universe had "zero" size, according to Einstein. The time between then and now is the age of the universe. There is one caveat to keep in mind that affects the certainty of the age determination: we assume that the universe is flat, which is well supported by WMAP and other data. If we relax this assumption within the allowed range, the uncertainty increases to a bit over 2%. However, theorists have long known that a nearly-flat universe is very difficult to produce, whereas inflation naturally predicts a flat universe.
The expansion age measured by WMAP is larger than the oldest globular clusters, so the Big Bang theory has passed an important test using data independent of the type collected by WMAP. If the expansion age measured by WMAP had been smaller than the oldest globular clusters, then there would have been something fundamentally wrong about either the Big Bang theory or the theory of stellar evolution. Either way, astronomers would have needed to rethink many of their cherished ideas. But our current estimate of age fits well with what we know from other kinds of measurements.
Is the Universe Infinite?
The shape of the universe is determined by a struggle between the momentum of expansion and the pull of gravity. The rate of expansion is expressed by the Hubble Constant, Ho, while the strength of gravity depends on the density and pressure of the matter in the universe. If the pressure of the matter is low, as is the case with most forms of matter we know of, then the fate of the universe is governed by the density. If the density of the universe is less than the "critical density" which is proportional to the square of the Hubble constant, then the universe will expand forever. If the density of the universe is greater than the "critical density", then gravity will eventually win and the universe will collapse back on itself, the so called "Big Crunch". However, the results of the WMAP mission and observations of distant supernova have suggested that the expansion of the universe is actually accelerating which implies the existence of a form of matter with a strong negative pressure, such as the cosmological constant. This strange form of matter is also sometimes referred to as the "dark energy". If dark energy in fact plays a significant role in the evolution of the universe, then in all likelihood the universe will continue to expand forever.
GEOMETRY OF THE UNIVERSE
The density of the universe also determines its geometry. If the density of the universe exceeds the critical density, then the geometry of space is closed and positively curved like the surface of a sphere. This implies that initially parallel photon paths converge slowly, eventually cross, and return back to their starting point (if the universe lasts long enough). If the density of the universe is less than the critical density, then the geometry of space is open, negatively curved like the surface of a saddle. If the density of the universe exactly equals the critical density, then the geometry of the universe is flat like a sheet of paper. Thus, there is a direct link between the geometry of the universe and its fate.
The simplest version of the inflationary theory, an extension of the Big Bang theory, predicts that the density of the universe is very close to the critical density, and that the geometry of the universe is flat, like a sheet of paper. That is the result confirmed by the WMAP science.
MEASUREMENTS FROM WMAP
The WMAP spacecraft can measure the basic parameters of the Big Bang theory including the geometry of the universe. If the universe were open, the brightest microwave background fluctuations (or "spots") would be about half a degree across. If the universe were flat, the spots would be about 1 degree across. While if the universe were closed, the brightest spots would be about 1.5 degrees across.
Recent measurements (c. 2001) by a number of ground-based and balloon-based experiments, including MAT/TOCO, Boomerang, Maxima, and DASI, have shown that the brightest spots are about 1 degree across. Thus the universe was known to be flat to within about 15% accuracy prior to the WMAP results. WMAP has confirmed this result with very high accuracy and precision. We now know that the universe is flat with only a 2% margin of error.
What is a Cosmological Constant?
Einstein first proposed the cosmological constant (not to be confused with the Hubble Constant) usually symbolized by the greek letter "lambda" (Λ), as a mathematical fix to the theory of general relativity. In its simplest form, general relativity predicted that the universe must either expand or contract. Einstein thought the universe was static, so he added this new term to stop the expansion. Friedmann, a Russian mathematician, realized that this was an unstable fix, like balancing a pencil on its point, and proposed an expanding universe model, now called the Big Bang theory. When Hubble's study of nearby galaxies showed that the universe was in fact expanding, Einstein regretted modifying his elegant theory and viewed the cosmological constant term as his "greatest mistake".
Many cosmologists advocate reviving the cosmological constant term on theoretical grounds. Modern field theory associates this term with the energy density of the vacuum. For this energy density to be comparable to other forms of matter in the universe, it would require new physics: the addition of a cosmological constant term has profound implications for particle physics and our understanding of the fundamental forces of nature.
The main attraction of the cosmological constant term is that it significantly improves the agreement between theory and observation. The most spectacular example of this is the recent effort to measure how much the expansion of the universe has changed in the last few billion years. Generically, the gravitational pull exerted by the matter in the universe slows the expansion imparted by the Big Bang. Very recently it has become practical for astronomers to observe very bright rare stars called supernova in an effort to measure how much the universal expansion has slowed over the last few billion years. Surprisingly, the results of these observations indicate that the universal expansion is speeding up, or accelerating! While these results should be considered preliminary, they raise the possibility that the universe contains a bizarre form of matter or energy that is, in effect, gravitationally repulsive. The cosmological constant is an example of this type of energy. Much work remains to elucidate this mystery!
There are a number of other observations that are suggestive of the need for a cosmological constant. For example, if the cosmological constant today comprises most of the energy density of the universe, then the extrapolated age of the universe is much larger than it would be without such a term, which helps avoid the dilemma that the extrapolated age of the universe is younger than some of the oldest stars we observe! A cosmological constant term added to the standard model Big Bang theory leads to a model that appears to be consistent with the observed large-scale distribution of galaxies and clusters, with WMAP's measurements of cosmic microwave background fluctuations, and with the observed properties of X-ray clusters.
WMAP AND THE COSMOLOGICAL CONSTANT
By characterizing the detailed structure of the cosmic microwave background fluctuations, WMAP is able to accurately determine the basic cosmological parameters, including the cosmological constant, to better than 5%.
Understanding the Evolution of Life in the Universe
HOW DID THE UNIVERSE START AND EVOLVE?
WMAP found that the universe is 13.7 billion years old. The universe began with an unimaginably enormous density and temperature. This immense primordial energy was the cauldron from whence all life arose. Elementary particles were created and destroyed by the ultimate particle accelerator in the first moments of the universe.
There was matter and there was antimatter. When they met, they annihilated each other and created light. Somehow, it seems that there was a tiny fraction more matter than antimatter, so when nature took its course, the universe was left with some matter, no antimatter, and a tremendous amount of light. Today, WMAP measures that there is more than a billion times more light than matter.
WE AREN'T MADE OF HYDROGEN!
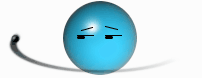
WMAP determined that about 4.6% of the mass and energy of the universe is contained in atoms (protons and neutrons). All of life is made from a portion of this 4.6%.
The only chemical elements created at the beginning of our universe were hydrogen, helium and lithium, the three lightest atoms in the periodic table. These elements were formed throughout the universe as a hot gas. It's possible to imagine a universe where elements heavier than lithium would never form and life never develop. But that is not what happened in our universe.
We are carbon-based life forms. We are made of and drink water (H2O). We breathe oxygen.
Carbon and oxygen were not created in the Big Bang, but rather much later in stars. All of the carbon and oxygen in all living things are made in the nuclear fusion reactors that we call stars. The early stars are massive and short-lived. They consume their hydrogen, helium and lithium and produce heavier elements. When these stars die with a bang they spread the elements of life, carbon and oxygen, throughout the universe. New stars condense and new planets form from these heavier elements. The stage is set for life to begin. Understanding when and how these events occur offer another window on the evolution of life in our universe.
WMAP determined that the first stars in the universe arose only about 400 million years after the Big Bang. But what made the stars?
THINGS THAT GO BUMP IN THE NIGHT.
![]() |
Quantum Fluctuations are the random nature of matter's state of existence or nonexistence. At these incredibly small sub-atomic scales, the state of reality is fleeting, changing from nanosecond to nanosecond. |
The motor for making stars (and galaxies) came early and was very subtle. Before the completion of the first fraction of a second of the universe, sub-atomic scale activity, tiny "quantum fluctuations", drove the universe towards stars and life. With the sudden expansion of a pinhead size portion of the universe in a fraction of a second, random quantum fluctuations inflated rapidly from the tiny quantum world to a macroscopic landscape of astronomical proportions. Why do we believe this? Because the microwave afterglow light from the Big Bang has an extraordinarily uniform temperature across the sky. There has not been time for the different parts of the universe to come into an equilibrium with each other *unless* the regions had exponentially inflated from a tiny patch. The only way the isotropy (uniformity) could have arisen is if the different regions were in thermal equilibrium with each other early in the history of the universe, and then rapidly inflated apart. WMAP has verified that other predictions from the inflation theory also appear to be true..
As the universe inflated, the tiny quantum fluctuations grew to become tiny variations in the amount of matter from one place to another. A tiny amount is all it takes for gravity to do its thing. Gravity is one of the basic forces of nature and controls the evolution of the large scale structure of the universe. Without gravity there would be no stars or planets, only a cold thin mist of particles. Without the variations in the particle soup initiated by the quantum fluctuations, gravity could not begin to concentrate tiny amounts of matter into even larger amounts of matter. The end result of the pull of gravity: galaxies, stars and planets. The fluctuations, mapped in detail by the WMAP mission, are the factories and cradles of life.
THE RECIPE FOR LIFE REQUIRES A DELICATE BALANCE OF COSMIC INGREDIENTS.
The differences in the early soup of universe particles were very small, so large scale changes take time to manifest themselves. What if our universe had only lasted for a second, or a year, or one million years? The age of the universe is controlled by the basic rules that govern matter, energy, and time. We needed almost 13.7 billion years to evolve and come to recognize this fact.
How long the universe lasts and how it evolves depends on its total energy and matter content. A universe with enormously more matter than ours would rapidly collapse back under its own gravity well before life could form. A very long lived universe might not have enough mass for stars to ever form. In addition, WMAP has confirmed the existence of a dark energy that acts like an anti-gravity, driving the universe to accelerate its expansion. Had the dark energy dominated earlier, the universe would have expanded too rapidly to support the development of life. Our universe seems to have Goldilocks properties: not too much and not too little -- just enough mass and energy to support the development of life.
IS THERE OTHER INTELLIGENT LIFE IN THE UNIVERSE?
We don't know whether or not there is other intelligent life in the universe. There is no reason there shouldn't be. We know by our own existence that the universe is conducive to life. But there are many hurdles to overcome for intelligent life to form, and many threats to its continued existence once it does form. Life constantly faces the prospect of extinction. Life requires energy, water, and carbon; an environmental disaster that removes water, dooms life. Other environment disasters threaten. On Earth we have had huge meteor impacts that are believed to have caused mass extinctions. The harsh radiation of space is blocked only by Earth's atmosphere and magnetic field. Environmental instabilities cause ice ages. One day, billions of years from now, our Sun will burn out. Other, heavier stars end their lives in explosions called supernovae; the blast and radiation from a nearby supernova could destroy all life on Earth.
The dark energy will inexorably stretch the universe into an icy cold end. Since we don't know what the dark energy is, this might be wrong, but no less deadly depending on how the nature of the dark energy changes.
Many people are engaged in efforts to detect life in the universe. There are two strategies: we look for it, or it finds us. Perhaps a middle ground would be if we detected signals coming from life elsewhere in the universe. The Search for Extraterrestrial Intelligence (SETI) program pioneered searches for life. WMAP itself is, in a small way, a mini-SETI experiment, since it constantly scans the skies over a wide range of microwave frequencies. WMAP was not optimized to search for life. Other efforts are (have been). Some day, we may know for sure whether we are alone in the universe. In the meantime the search goes on, as we also try to understand the universe and how it may be conducive to life.
By detecting and measuring the density fluctuations in the cosmic microwave background using the WMAP space mission we are learning about the early universe; and we begin to understand the basic ingredients that make life possible. In the future, we would like to enhance these efforts with other missions, such as NASA's Einstein Inflation Probe, which would strive to detect the gravity disturbances from the era when the universe originally inflated. This passionate search for knowledge is characteristic of human life.
What is the Ultimate Fate of the Universe?
Just as Robert Frost imagined two possible fates for the Earth in his poem, cosmologists envision two possible fates for the universe:
- Endless expansion
- The “Big Crunch”
The evolution of the universe is determined by a struggle between the momentum of expansion and the pull (or push!) of gravity. The current rate of expansion is measured by the Hubble Constant, while the strength of gravity depends on the density and pressure of the matter in the universe. If the pressure of the matter is low, as is the case with most forms of matter we know of, then the fate of the universe is governed by the density.
If the density of the universe is less than the critical density, then the universe will expand forever, like the green or blue curves in the graph above. Gravity might slow the expansion rate down over time, but for densities below the critical density, there isn’t enough gravitational pull from the material to ever stop or reverse the outward expansion. This is also known as the “Big Chill” or “Big Freeze” because the universe will slowly cool as it expands until eventually it is unable to sustain any life.
If the density of the universe is greater than the critical density, then gravity will eventually win and the universe will collapse back on itself, the so called “Big Crunch”, like the graph's orange curve. In this universe, there is sufficient mass in the universe to slow the expansion to a stop, and then eventually reverse it.
Recent observations of distant supernova have suggested that the expansion of the universe is actually accelerating or speeding up, like the graph's red curve, which implies the existence of a form of matter with a strong negative pressure, such as the cosmological constant. This strange form of matter is also sometimes referred to as the “dark energy”. Unlike gravity which works to slow the expansion down, dark energy works to speed the expansion up. If dark energy in fact plays a significant role in the evolution of the universe, then in all likelihood the universe will continue to expand forever.
There is a growing consensus among cosmologists that the total density of matter is equal to the critical density, so that the universe is spatially flat. Approximately 3/10 of this is in the form of a low pressure matter, most of which is thought to be “non-baryonic” dark matter, while the remaining 7/10 is thought to be in the form of a negative pressure “dark energy”, like the cosmological constant. If this is true, then dark energy is the major driving force behind the fate of the universe and it will expand forever exponentially.
MEASUREMENTS FROM WMAP
The WMAP satellite measures the basic parameters of the Big Bang theory including the fate of the universe. The results suggest the geometry of the universe is flat and will expand forever. Further study of the dark energy with future experiments and space missions is needed to understand its nature and effect on the rate of future expanison.